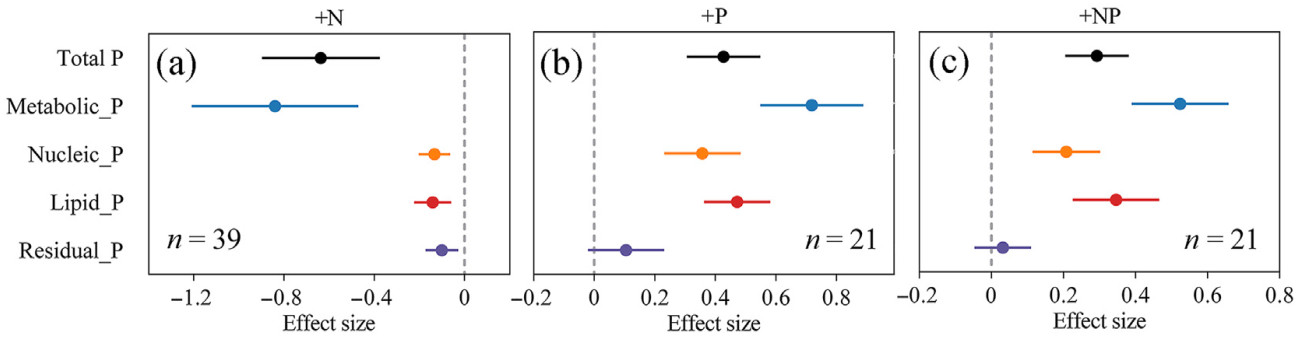
Citation: | Qingquan Meng, Zhijuan Shi, Zhengbing Yan, Hans Lambers, Yan Luo, Wenxuan Han. Independent and interactive effects of N and P additions on foliar P fractions in evergreen forests of southern China[J]. Forest Ecosystems, 2025, 12(1): 100265. DOI: 10.1016/j.fecs.2024.100265 |
Fertilization or atmospheric deposition of nitrogen (N) and phosphorus (P) to terrestrial ecosystems can alter soil N (P) availability and the nature of nutrient limitation for plant growth. Changing the allocation of leaf P fractions is potentially an adaptive strategy for plants to cope with soil N (P) availability and nutrient-limiting conditions. However, the impact of the interactions between imbalanced anthropogenic N and P inputs on the concentrations and allocation proportions of leaf P fractions in forest woody plants remains elusive. We conducted a meta-analysis of data about the concentrations and allocation proportions of leaf P fractions, specifically associated with individual and combined additions of N and P in evergreen forests, the dominant vegetation type in southern China where the primary productivity is usually considered limited by P. This assessment allowed us to quantitatively evaluate the effects of N and P additions alone and interactively on leaf P allocation and use strategies. Nitrogen addition (exacerbating P limitation) reduced the concentrations of leaf total P and different leaf P fractions. Nitrogen addition reduced the allocation to leaf metabolic P but increased the allocation to other fractions, while P addition showed opposite trends. The simultaneous additions of N and P showed an antagonistic (mutual suppression) effect on the concentrations of leaf P fractions, but an additive (summary) effect on the allocation proportions of leaf P fractions. These results highlight the importance of strategies of leaf P fraction allocation in forest plants under changes in environmental nutrient availability. Importantly, our study identified critical interactions associated with combined N and P inputs that affect leaf P fractions, thus aiding in predicting plant acclimation strategies in the context of intensifying and imbalanced anthropogenic nutrient inputs.
Phosphorus (P) is an essential element for sustaining life on Earth. It plays a crucial role in the synthesis of nucleic acids and membranes, as well as in the regulation of enzyme activities, thereby exerting a significant impact on fundamental biological processes such as photosynthesis and respiration (Lambers, 2022). Meanwhile, P is considered to be a major limiting element for plant activity and primary productivity in forest ecosystems (Elser et al., 2007; Hawkesford et al., 2023; Li et al., 2016). Recent research has emphasized the potential significance of leaf P allocation among various functional components in providing insights into plant adaptation to P limitation (Hidaka and Kitayama, 2011; Lambers, 2022; Veneklaas et al., 2012).
To adapt to low soil P availability, plants must strike a balance in allocating P to different leaf P fractions (Hayes et al., 2022; Wen et al., 2023), involving the prioritization of leaf P fractions that are essential for vital functions such as photosynthesis, while reducing allocation to secondary functions like structural roles. Leaf P can be divided into four fractions using chemical extraction methods, including metabolic P, lipid P, nucleic acid P and residual P. Metabolic P refers to inorganic P (Pi) and small P-containing metabolites (metabolite P); Pi is a soluble fraction located in the cytoplasm where it participates in metabolic activities, whereas excess Pi is stored in vacuoles to buffer the P concentration in the cytoplasm (Veneklaas et al., 2012). Metabolite P mainly comprises intermediates of carbon metabolism such as sugar phosphates, and these compounds play a crucial role in the Calvin cycle and glycolysis (Tsujii et al., 2023; Veneklaas et al., 2012; Wen et al., 2023). Lipid P is composed of phospholipids, which play a crucial role in membranes. Specifically, they are major components of both the plasmalemma and organelle membranes, especially the endoplasmic reticulum (Lambers, 2022). Nucleic acid P comprises biomolecules that are essential for life, including DNA which carries genetic information in living systems, and RNA which regulates protein turnover and synthesis (de Oliveira et al., 2018). Residual P is a fraction difficult to extract by chemical methods, including phosphorylated proteins and possibly some unidentified P-containing compounds (Hidaka and Kitayama, 2011; Mo et al., 2019).
Fertilization and atmospheric deposition have significantly increased N and P inputs into terrestrial ecosystems (Elser et al., 2007). Nitrogen inputs are more prevalent in terrestrial ecosystems than P inputs (Peñuelas et al., 2012, 2013); however, in specific regions, excessive application of P fertilization has led to elevated P inputs, which disrupt the equilibrium between N and P inputs (Cech et al., 2008). The imbalance between N and P inputs can result in N limitation, P limitation, or both (Peñuelas et al., 2013). Global N modeling projections indicated that, in the coming decades, southern China will be among the areas most significantly affected by N deposition (Galloway et al., 2008). Evergreen forests are the predominant vegetation type in southern China, and their primary productivity is usually considered limited by P (Shi et al., 2024); moreover, the high N deposition in southern China is assumed to exacerbate this P limitation and potentially alter plant nutrient utilization strategies in these evergreen forest ecosystems (Yuan and Chen, 2015b). Researchers have sought to investigate how plant nutrient use strategies respond to N and P inputs in this area by analyzing leaf P fractions. However, from the limited number of available studies, the concentrations and allocations of leaf P fractions exhibit inconsistent trends - either increasing, decreasing, or showing no marked change with nutrient addition, presenting incongruent models of P fraction allocation (Mo et al., 2019; Yu et al., 2022). So far, the influence of N and P additions on the concentrations and allocation proportions of leaf P fractions remains not fully understood. Therefore, examining the response of evergreen forests to unbalanced N and P inputs in southern China can offer valuable insights into nutrient cycling in P-limited areas and inform strategies for plant nutrient utilization in the context of increasing and imbalanced anthropogenic nutrient inputs.
Nitrogen addition either reduces leaf P fractions concentrations or has no significant effect (Su et al., 2024; Yu et al., 2022). Phosphorus addition and combined N and P additions typically increased leaf P fractions concentrations, though some studies reported no significant effect (Mo et al., 2019; Zhang et al., 2021). Research on leaf P fraction concentrations suggests that as natural soil P availability decreases and P limitation increases, the residual P concentration in plant leaves remains relatively constant; however, metabolic P, lipid P, and nucleic acid P concentrations show a declining trend, which may be related to the release of Pi from vacuoles, lipid remodeling, and reduced protein synthesis (Gao et al., 2022a; Hidaka and Kitayama, 2011; Yan et al., 2019). Therefore, we hypothesized that N addition (exacerbating P limitation) would decrease the leaf total, metabolic, lipid, and nucleic acid P concentrations; conversely, P addition (weakening P limitation) would trend in the opposite direction. Additionally, N and P usually were added at low ratios in actual combined N and P-addition experiments (Crowther et al., 2019; Garbowski et al., 2023; Widdig et al., 2020). Such treatment protocols could enhance the effect of P addition; therefore, we hypothesize that the effect of combined N and P addition is similar to that of P addition alone (Hypothesis 1).
In environments with severe P deficiency, certain plants adopt a strategy to maintain basic leaf physiological functions, including protein synthesis turnover and photosynthesis, by reducing overall metabolic activity; this adaptive approach allows them to reduce their overall demand for leaf P (Han et al., 2022; Hidaka and Kitayama, 2011; Mo et al., 2019; Warren, 2011; Zhang et al., 2021). Several studies have investigated the variation in leaf P fraction concentrations with soil P availability. A consistent trend has emerged: as soil P availability decreases, plants reduce the allocation to metabolic P while increasing the allocation to nucleic acid P and lipid P (Gao et al., 2022a, 2022b; Hidaka and Kitayama, 2011). Nitrogen addition typically does not affect the allocation of leaf P fractions, though some studies have observed an increase in the allocation to nucleic acid P and lipid P (Mo et al., 2019; Yu et al., 2022). In contrast, P addition and co-addition of N and P usually cause an increase in the allocation of metabolic P fractions (Hayes et al., 2022; Yu et al., 2022). Based on these findings, it is hypothesized that N addition (exacerbating P limitation) reduces the allocation to leaf metabolic P while increasing the allocation of P to leaf nucleic acid and lipid P; conversely, leaf P allocation will exhibit the opposite pattern under P addition and combined N and P addition (weakening P limitation) (Hypothesis 2).
As two essential mineral nutrients for plants, N and P are functionally coupled in biological systems, from cells to ecosystems (Ågren et al., 2012; Han et al., 2005; Reich and Oleksyn, 2004; Sterner and Elser, 2002). Previous studies have demonstrated that interactions between N and P are widespread in the biosphere. For example, at the molecular level, N-P interactions influence the expression of genes that are responsive to P starvation and nitrate transport (Hu and Chu, 2020). At the cell level, N-P interactions influence the concentrations and allocations of leaf P fractions (Zhang et al., 2021). At the individual level, N-P interactions regulate plant growth rates, stoichiometry, and nutrient uptake (Yan et al., 2015). Finally, at the ecosystem level, N-P interactions affect ecosystem productivity (Elser et al., 2007). To predict plant responses to imbalanced N and P inputs, it is crucial to determine whether plant growth responses to N and P addition are interdependent; if they are, it is important to identify the primary type of interaction. The combined N-P addition may result in additive, antagonistic, or synergistic effects. When N and P are co-added, the positive effects of P additions in P-limited systems may be diminished or entirely negated by N additions, suggesting the potential for either additive or antagonistic effects (Li et al., 2016; Su et al., 2014). In contrast, synergistic effects tend to occur in situations where systems are co-limited by N and P (Harpole et al., 2011). Plant biomass shows significant synergistic responses to N and P additions in terrestrial ecosystems (Elser et al., 2007; Harpole et al., 2011; Jiang et al., 2019); however, for most P-related metrics, the impact tends to be additive; this is observed in metrics such as total leaf P concentration, leaf N-to-P ratio (N: P), concentrations of total soil P, available soil P, and microbial biomass P (Jiang et al., 2019; Yuan and Chen, 2015a; Yue et al., 2018). Currently, there is insufficient research on the interactive effects of N and P additions on the concentrations and allocation proportions of leaf P fractions. We hypothesize that similar to other P-related metrics, the impact of combined N and P addition on the concentrations and allocation proportions of different leaf P fractions is additive (Hypothesis 3).
We carried out a thorough meta-analysis utilizing the data that was accessible from peer-reviewed case studies. The study had three specific objectives: (1) to clarify the effects of N and P additions on the concentrations of leaf P fractions, both individually and combined; (2) to determine the allocation proportions of leaf P fractions impacted by N and P additions individually and combined; and (3) to examine the interactive effects of N and P co-addition on both the concentrations and allocation proportions of different leaf P fractions.
Literature retrieval was conducted using the Web of Science and China National Knowledge Infrastructure until January 2024. The search used the following terms: ("N fertilization", "N addition", "N deposition", "N enrichment", and "N limitation"; "P fertilization", "P addition", "P deposition", "P enrichment", and "P limitation"; "nutrient addition", "nutrient enrichment", and "nutrient limitation") in combination with ("leaves", "leaf") and ("P fraction", "P allocation"). Furthermore, potential data collection was conducted by reviewing the references cited in the retrieved literature.
The criteria for paper selection were as follows:
(1) All studies were based on evergreen forests in southern China;
(2) Leaves must be mature and green, not young or senescent;
(3) Sampling must be based on natural field conditions, not greenhouse or indoor experiments;
(4) Leaves must be freeze-dried before measuring P fractions, not air-dried;
(5) The paper must contain all four required fractions or more, as needed for this study;
(6) The literature should include both controls and nutrient-addition treatments conducted under identical conditions;
(7) Data presented in the literature should consist of mean values and standard deviation (or standard error).
We obtained the required data directly from tables and supplementary files, or indirectly extracted from figures using GetData (version 2.20). Finally, our dataset includes 39 instances of N addition, 21 instances of P addition, and 21 instances of N and P co-addition for 17 woody evergreen species from southern China (Supplementary data). Additionally, data of leaf N: P ratio were also collected, given that this parameter is commonly used to indicate N and P limitation types that affect plant growth. When the leaf N: P ratio is greater than 16, the growth of plants is often limited by P (Han et al., 2013; Koerselman and Meuleman, 1996). According to these criteria of leaf N: P, the growth of 80% woody plant species in the field-experiment studies (the control groups) could be assumed to be limited by P in their natural habitats (Supplementary data, Fig. S1).
The Shapiro-Wilk test was used for the assessment of the normality of the data before the statistical analyses. To improve data normality, we applied a logarithmic transformation, and then analyzed the correlations between the concentrations and allocation proportions of different leaf P fractions using standardized major axis regression with the 'smart' package in R 4.1.3 (R Development Core Team, 2022).
Initially, missing standard deviations in the data were calculated using the Bracken (1992) method. The 'metagear' package in R was then used to complete the missing data. The meta-analysis used the natural logarithm of the response ratio (RR) (Hedges et al., 1999) to evaluate the impacts of N addition, P addition, and combined N and P addition on total leaf P concentration, different P fraction concentrations, and the allocation proportions of different P fractions. The calculation formula was as follows:
RR=ln(XeXCK)=ln(Xe)−ln(XCK) | (1) |
The RR is an indicator of the impact of the experimental treatment on the target variable and is the ratio of the mean of the selected variable in the treatment group (Xe) to that in the control group (XCK).
The detailed calculation of the variance (vi) and weight (wi) of each RR, and the overall effect size (RR) were described in the Supplementary Information (Text S1).
Our study's robustness to publication bias was assessed using Egger's test (Egger et al., 1997) and Rosenberg's fail-safe factor (Rosenberg, 2005). All calculations performed in R used the 'metafor' package. The results indicated that most variables did not exhibit publication bias (Table S1); moreover, for certain variables where potential publication bias was observed, it is unlikely to have impacted the outcomes, as Rosenberg's fail-safe factor considerably exceeded 5N+10 (N, the number of observations) (Table S2).
We employed Hedges' d to compute the main effects and interactive effects between N and P addition on the concentrations and allocation proportions of leaf P fractions (Gurevitch and Hedges, 2001). The main effects were determined by comparing the net effects of a single addition in the presence and absence of the second addition; this resembles testing for main effects in the analysis of variance, emphasizing one factor's unique influence at different levels of another factor (Crain et al., 2008). For each observed value, the formulas for calculating the effects of the two driving factors, N addition (d+N) and P addition (d+P), and their interactive effect (d+NP), were as follows:
d+Ni=(X+N−XCK)+(X+NP−XCK)−(X+P−XCK)2s×J | (2) |
d+Pi=(X+P−XCK)+(X+NP−XCK)−(X+N−XCK)2s×J | (3) |
d+NPi=(X+NP−XCK)−(X+N−XCK)−(X+P−XCK)s×J | (4) |
where XCK, X+N, X+P, and X+NP represent the mean values of the control, N addition, P addition, and their combination for observation i, while s denotes the pooled standard deviation, and J is a correction term. The detailed calculation of i, s, J, and the overall weighted mean of Hedges' d were described in the Supplementary Information (Text S2).
The calculation for the 95% confidence interval (CI) was determined using the standard normal distribution's two-tailed critical value (Cα/2) and the standard error of dt (st) (Zhou et al., 2016). Based on the 95% CI of the interactive effect, the types of interaction were classified as additive (if the 95% CI of d+NP overlapped with 0), synergistic (if the sign of d+NP matched the sum of d+N and d+P), or antagonistic (if the sign of d+NP opposed the sum of d+N and d+P) (Fig. S2) (Crain et al., 2008; Jiang et al., 2021; Zhou et al., 2016).
Nitrogen addition significantly decreased the concentration of leaf total P and all P fractions (P < 0.05); in contrast, P addition significantly increased the concentration of leaf total P and all P fractions, except residual P (P < 0.05). The results of combined N and P additions were consistent with P addition (Fig. 1a–c). Analysis of the different P fraction concentrations in the leaves also revealed a positive correlation among them (Fig. 2a–f).
Nitrogen addition significantly decreased the proportion of leaf metabolic P (P < 0.05) and significantly increased the proportions of other fractions (P < 0.05). The results of combined N and P additions were consistent with those of P addition only; both significantly increased the proportion of leaf metabolic P, and significantly decreased the proportions of leaf nucleic acid P and residual P (P < 0.05) (Fig. 3a–c). Analysis of the allocation proportions of different leaf P fractions revealed a significant negative correlation between metabolic P and the proportions of other fractions, while a significant positive correlation existed among the other fractions (Fig. 4a–f).
The co-addition of N and P had an additive effect on leaf residual P concentration (Fig. 5e), but an antagonistic effect on concentrations of leaf total P and P fractions (Fig. 5a–d). The proportions of additive, synergistic, and antagonistic interactions varied for total P and concentrations of different leaf P fractions. The interaction between N and P co-addition had predominantly additive effects on leaf total P and residual P concentrations (Fig. 5f), while the interaction had mainly antagonistic effects on metabolic P, nucleic acid P, and lipid P concentrations (Fig. 5f).
The co-addition of N and P had an additive effect on leaf nucleic acid P and lipid P allocations, but it had an antagonistic effect on leaf metabolic P and residual P allocations (Fig. 6a–d). Additive effects were the primary factor influencing the allocation impact on different leaf P fractions, accounting for 61.9% to 76.2% of the total number of studies (Fig. 6e).
When N and P were added separately, they had opposite effects on the concentrations and allocations of leaf P fractions. However, the results of the combined N and P additions were similar to those of P addition alone, suggesting that P addition plays a predominant role in influencing both the concentrations and allocation of leaf P fractions. This is because, in our dataset (as detailed in the Supplementary data), N and P were co-added in equal mass (i.e., with a molar ratio of 31:14), which significantly reduced the leaf N: P, consequently accentuating the influence of P addition. Given the importance of P fraction concentrations and allocation proportions under P limiting conditions (Hidaka and Kitayama, 2011), here we only discuss the impact of N addition (exacerbating P limitation) on the concentrations and allocation proportions of leaf P fractions. The trend reversed when P limitation weakened (P addition, and combined N-P addition) (Figs. 1 and 3).
Our findings support Hypothesis 1, which stated that N addition (exacerbating P limitation, Fig. S3) results in a reduction of total leaf P concentration (Fig. 1a), consistent with earlier research (Jiang et al., 2019), and decreases the concentrations of all fractions. Nitrogen addition (exacerbating P limitation, Fig. S3) reduced metabolic P levels (Fig. 1a). A decrease in inorganic P concentration may be the primary cause (Su et al., 2024). Inorganic P is a labile fraction functioning in the cytoplasm for metabolic processes. Under P-limiting conditions, inorganic P stored in the vacuoles is depleted and utilized for other physiological processes (Liu et al., 2016; Xu et al., 2019).
Nitrogen addition also decreased the concentration of lipid P (Fig. 1a). This reduction is attributed to lipid remodeling, a process that often occurs in environments with severe P deficiency (Lambers et al., 2012). In this process, non-P lipids such as sulfolipids and glycolipids replace phospholipids, which are degraded, making phosphate available for other cellular functions (Härtel et al., 2000; Lambers et al., 2012; Yang et al., 2021).
Nitrogen addition resulted in a decreased concentration of nucleic acid P (Fig. 1a). Nucleic acids consist of sugar molecules, bases, and phosphate groups; the main component is ribosomal RNA (rRNA). In environments with limited resources for phosphate, cells may not be able to provide enough phosphate for rRNA synthesis, leading to a decrease in ribosomal abundance (Lambers, 2022).
Furthermore, a significant decrease in residual P concentration was also observed with N addition (Fig. 1a). Residual P mainly consists of phosphorylated proteins. This reduction may occur because, under severe P-deficient conditions, phosphatase can dephosphorylate phosphorylated proteins, releasing inorganic P for reuse by the plant (Yang et al., 2020).
Our findings support Hypothesis 2 that N addition (exacerbating P limitation, Fig. S3) led to a decrease in the allocation proportion of leaf metabolic P and an increase in the allocation proportions of leaf nucleic acid and lipid P (Fig. 3a). Although the concentrations of all leaf P fractions decreased with the N addition, the concentration of leaf metabolic P decreased to a much greater extent than the other P fractions. This may have led to a decrease in the allocation proportion of leaf metabolic P and an increase in the allocation proportions of other leaf P fractions (Fig. 3a). The decrease in leaf metabolic P allocation was primarily attributed to the fact that plant growth was P-limited (as detailed in the Supplementary data), which is further exacerbated by N addition, resulting in the depletion of inorganic P in leaf vacuoles (Veneklaas et al., 2012; Suriyagoda et al., 2023). The observed increase in leaf nucleic acid P is likely due to plants prioritizing limited P resources towards ribosomal RNA (rRNA) synthesis to sustain protein synthesis for growth and reproduction (Veneklaas et al., 2012). Furthermore, N addition led to an increase in the partitioning to leaf lipid P. The endoplasmic reticulum accounts for over 60% of phospholipid mass in various cell types which serves as a pathway for intracellular substance transport and the site of intracellular reactions of many enzymes (Benning, 2009; Lagace and Ridgway, 2013). The increased partitioning to leaf lipid P might result in faster rates of substance transport and enzyme reactions. This is beneficial for plant adaptation to P limitation.
An increase in leaf residual P (mainly phosphorylated proteins) allocation was also observed with the addition of N (Fig. 3a). This may be because plants allow acclimation to P deficiency by increasing the level of protein phosphorylation (Zhang et al., 2023), which involves the regulations of cell division, metabolism, intracellular signal transduction, nutrient uptake and transport, to maintain plant growth and development (Li et al., 2015; van Bentem and Hirt, 2007; van den Berg et al., 2016).
Our findings only partially support Hypothesis 3. The additive effect of combined additions of N and P on the leaf P fraction was observed solely in the allocation proportions, not in the concentrations (Fig. 5, Fig. 6). The combined N and P additions on leaf P fraction concentrations did not exhibit common additive effects; instead, they showed antagonistic and negative effects (Fig. 5a–d). The outcome of this study is comparable to the findings of Paul et al. (2023). On the one hand, the antagonistic effect may be attributed to the study mainly including samples from the southern regions of China, where soils have experienced significant leaching and weathering of parent materials, resulting in relatively low available P. Concurrently, N deposition induces soil acidification (Lu et al., 2014), which favors the sorption of P by iron and aluminum, thus reducing P availability (Weil and Brady, 2017). On the other hand, N addition has been reported to promote plant growth and increase leaf biomass by 13.4% (Feng et al., 2023), thus decreasing the concentration of P in the leaves via a dilution effect (Aerts et al., 2001).
The interactive effect between N and P co-addition had an additive effect on the allocation proportions of nucleic acid and lipid P (Fig. 6b and c), but there was a non-additive effect on the allocation proportions of metabolic and residual P under N and P co-addition (Fig. 6aand d). Nonetheless, additive effects predominated in all fractional allocations (61.9%–76.2%) (Fig. 6e), suggesting that additive effects between N and P may be common. The underlying mechanism may involve plants responding to changes in environmental nutrient availability by altering their allocation of P fractions. Our findings revealed opposite responses in the allocation of leaf P fractions under individual N vs P addition (Fig. 3a and b), which may neutralize each other's effects and result in the additive effect on leaf P-fraction allocation when both N and P are added (Fig. 6e). Previous research has also identified the prevalence of additive effects of N and P co-addition on several P-related metrics in terrestrial ecosystems (Jiang et al., 2019; Yuan and Chen, 2015a; Yue et al., 2018). This observation suggests that the effects of N and P co-additions on leaf P fraction allocation are relatively independent and do not interact with each other.
In response to the addition of N alone, the concentrations of P and its fractions in leaves decreased; the proportion of metabolic P (mainly metabolite P) decreased, while those of other fractions increased. This suggests that plants tended to reduce the overall demand for leaf P and adjust their allocation to different fractions in response to P limitation, at least for evergreen forests of southern China. Compared to N addition, an opposite trend emerged when we added P alone, or both N and P. Furthermore, the simultaneous addition of N and P shows antagonistic (concentrations) and additive effects (allocation proportions) on the leaf P fractions, which may help better predict the responses of forest productivity in the context of intensifying and imbalanced global anthropogenic nutrient inputs with improved forest biogeochemical models. Our results underscore the importance of P allocation strategies in woody plants' acclimation to changing environmental nutrient availability. Future research can also investigate the influence of other global change factors, e.g., water balance, on plant leaf P fractions, thus providing a more comprehensive understanding of allocation to leaf P fractions in plant response to environmental changes.
Qingquan Meng: Writing – original draft, Visualization, Methodology, Formal analysis, Data curation. Zhijuan Shi: Writing – review & editing. Zhengbing Yan: Writing – review & editing. Hans Lambers: Writing – review & editing. Yan Luo: Writing – review & editing. Wenxuan Han: Writing – review & editing, Supervision, Funding acquisition, Conceptualization.
The data in this article is attached to the supporting information, please contact the corresponding author if you need to use this data.
The authors declare that they have no known competing financial interests or personal relationships that could have appeared to influence the work reported in this paper.
We sincerely thank all the scientists whose data and work were included in this meta-analysis. We are also grateful to the handling editor and anonymous referees for their insightful comments and suggestions which have greatly improved our manuscript.
Supplementary data to this article can be found online at https://doi.org/10.1016/j.fecs.2024.100265.