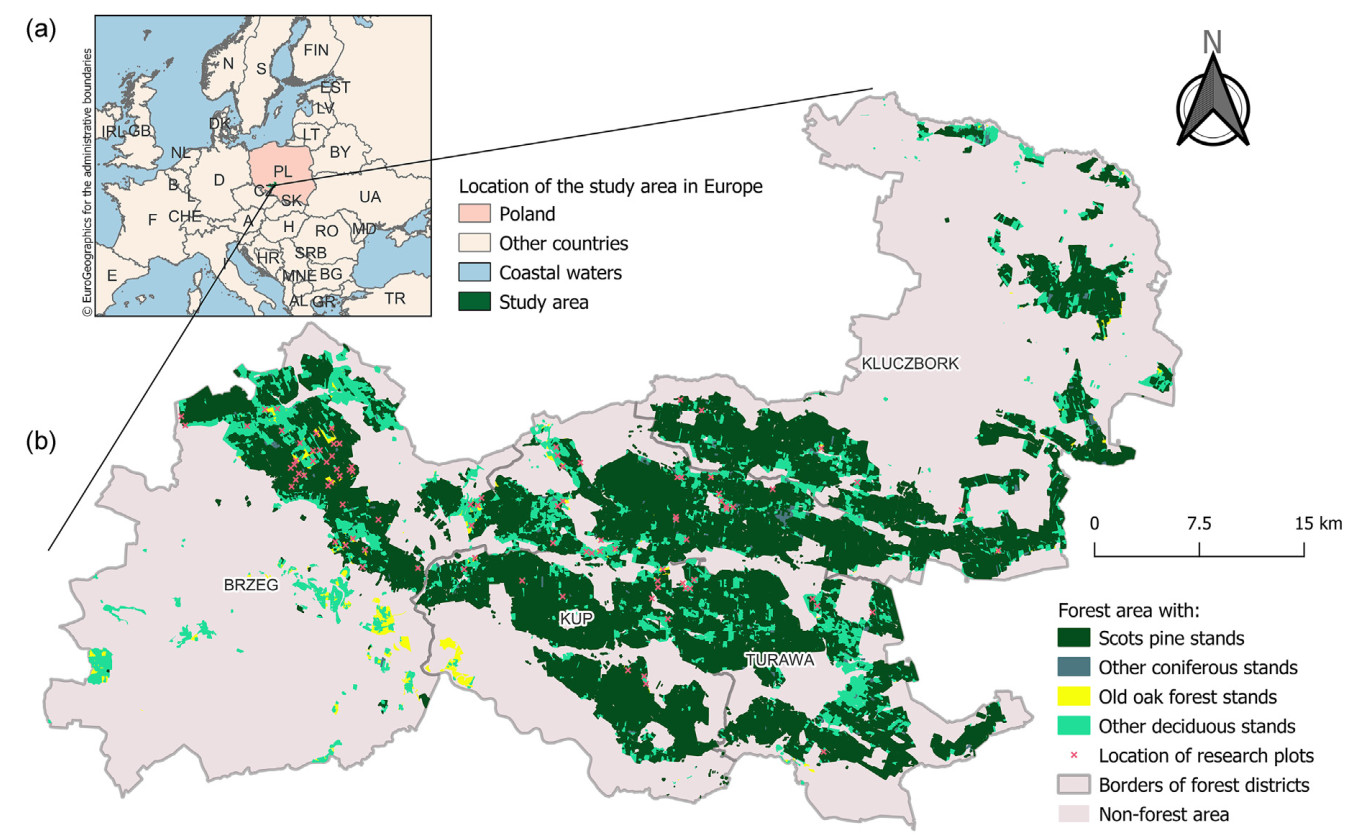
Citation: | Ewa Stefańska-Krzaczek, Rafał Krzaczek, Natalia Mazurek, Damian Chmura. Variability and determinants of vascular plant species composition in patches of old managed oak forest stands dispersed within Scots pine monocultures[J]. Forest Ecosystems, 2024, 11(1): 100235. DOI: 10.1016/j.fecs.2024.100235 |
In the temperate zone of Europe, Scots pine forests are expected to occupy the poorest habitats which are unfavourable for deciduous trees. However, as a result of deforestation of the most fertile habitats and the preference for Pinus sylvestris in silviculture, pine forests have become the dominant feature of the landscape in Central Europe. As a result, the area of optimal habitat for deciduous forest flora has been significantly reduced. Nevertheless, remnant patches of deciduous forest persist as habitat islands within extensive pine forest complexes and may serve as important refugia for meso- and eutrophilic forest species. However, the factors that contribute to the variation in species composition of such habitat islands and their role in maintaining biodiversity remain poorly understood. This paper aims to fill this knowledge gap.
The studied deciduous (oak) stands exhibited a diverse vegetation, with species composition influenced by overstorey attributes, oak age, patch location, and the area and circularity of the deciduous forest. Species traits related to environmental variables included mainly preferences for closed or open forests, requirements for soil moisture, reaction, and fertility, and requirements for light conditions. The key message from our research is that oak islands are not simply patches of trees that are different from the surrounding area. Instead, they represent distinct plant communities that have developed to adapt to the prevailing environmental conditions by recruiting species with particular traits. The presence of oak islands within Scots pine monocultures is therefore important for increasing the diversity of the forest complex.
The most relevant recommendation from our research for forest management is to maintain as many patches of deciduous tree stands as possible within a single pine forest complex, as even small habitat islands can make a significant contribution to the biodiversity of the forest complex.
Forests cover 31% of the world's land area and are the most important habitats for terrestrial biodiversity (FAO and UNEP, 2020). Climatic conditions determine the variation in forest diversity and species composition on a global scale (Bouchard et al., 2024; Suzuki et al., 2006). At regional and local scales, within a given climatic zone, forest diversity is influenced by a variety of additional factors. Geomorphology, soil conditions, and microclimate play an important role in the variation of forest species composition (Bátori et al., 2023; Leuschner and Lendzion, 2009; Pielech et al., 2015; Sellan et al., 2019; Sewerniak and Puchałka, 2020). Furthermore, the structure of the tree stand is crucial for the composition of the understorey, as different tree species determine different conditions under the canopy (Augusto et al., 2003; Barbier et al., 2008; Tinya et al., 2021; Yu and Sun, 2013). Species composition also changes during forest succession (Hilmers et al., 2018) and depends on the continuity of the forest habitat (Matuszkiewicz et al., 2013; Nordén et al., 2014; Schmidt et al., 2014). In addition, the natural variability of tree stand age and composition is significantly altered by forest management practices (Burrascano et al., 2018; Dieler et al., 2017; Stefańska-Krzaczek et al., 2019). Moreover, fragmentation of forest vegetation and isolation of patches can also have a significant impact on the forest species composition (Echeverría et al., 2007; Jamoneau et al., 2011). The combination of many possible factors influencing a single forest complex makes it necessary to continuously study the formation and functioning of forests in order to understand their diversity. However, the results of all studies must have the same application: they should facilitate the development of solutions for sustainable forest management, with particular emphasis on maintaining biodiversity and forest ecosystem services (MacDicken et al., 2015; Oettel and Lapin, 2021; Siry et al., 2005). This is particularly important in the context of global climate change (De Frenne et al., 2021; Hansen et al., 2001; Vacek et al., 2023).
The most valuable forests are primary and old-growth forests which cover about a third (34%) of the world's forests (FAO and UNEP, 2020). However, the majority of forests in Europe are semi-natural, with primary and old-growth forests covering only 0.7% of the forest area (Sabatini et al., 2018). The largest area is covered by managed tree stands aged 21–60 years, and the proportion of stands older than 140 years is the lowest (Barbati et al., 2014; Vilén et al., 2012). Even the oldest managed stands are relatively young, as forest rotations rarely exceed 200 years and most tree species reach harvest age at around 100–150 years (Pach et al., 2018; Schütz et al., 2016). This suggests that managed stands are harvested and regenerated when the existing plant community reaches the optimal stage of succession which can last 300–400 years in forest habitats (Emborg, 1998; Hilmers et al., 2018). However, due to the very low cover of primary and old-growth forests (Barredo et al., 2021; Mikoláš et al., 2019; Sabatini et al., 2018), the largest habitat area for forest flora is available in managed forests, although these are usually age-class forests with simplified structural diversity (Bauhus et al., 2009; Burrascano et al., 2018). Subsequent age classes of managed forest stands make a special contribution to the diversity of forest complexes (Stefańska-Krzaczek et al., 2019; Ujházy et al., 2017), but the specific microclimate and diverse microhabitats develop only in forest stands of the oldest age classes (Máliš et al., 2023; Thompson et al., 2022). Therefore, the oldest forest stands usually represent the most developed (reference) patches of plant communities (Krzaczek and Stefańska-Krzaczek, 2022), even if they are not distinguished by the presence of specialised late seral species (Durak and Holeksa, 2015; Stefańska-Krzaczek et al., 2019). In order to achieve a balance between the use of forest resources and the need for forest conservation, further research is needed on the factors influencing the species composition of the oldest managed stands.
Coniferous forests in the European temperate zone are a natural component of the vegetation (Leuschner and Ellenberg, 2017). However, in a natural landscape they usually occupy nutrient-poor habitats where conditions are not favourable for the growth of deciduous trees (Matuszkiewicz, 2001, 2008). Significant deforestation of fertile habitats and the promotion of conifers in silviculture have led to a reversal in the proportions of deciduous and coniferous species in the forests of Central Europe. Pinus sylvestris L. is currently the dominant species in Central European lowland forests, while deciduous forests occupy a small area (Brus et al., 2012). This is an important issue for biodiversity, as deciduous forests provide habitats for the most specialised forest species (Hermy et al., 1999; Mölder et al., 2019; Schmidt et al., 2014; Stefańska-Krzaczek et al., 2016). Furthermore, the vegetation of deciduous forests in Central Europe is more diverse than that of coniferous forests. This is evidenced by the existence of a larger number of deciduous forest types than coniferous forest types (Leuschner and Ellenberg, 2017; Matuszkiewicz, 2001). Consequently, the conservation of most forest flora resources and forest plant communities is primarily linked to the maintenance of deciduous forests in good condition. This, in turn, highlights the need to identify the factors that determine deciduous forest diversity.
As a result of deforestation of fertile habitats, some deciduous forests have survived as small patches within large pine complexes that have remained in poor habitats. Due to the relatively broad tolerance of oak to environmental conditions, it may be a common component of patches of deciduous stands scattered within Scots pine monocultures. The pedunculate oak Quercus robur L. and the sessile oak Quercus petraea (Matt.) Liebl. form different natural types of deciduous forests in Central Europe (Mölder et al., 2019; Pividori et al., 2016; Spiecker, 2021). They are important components of acidophilous oak forests, thermophilous oak forests, oak-hornbeam forests, and riparian forests (Kącki et al., 2013, 2016; Leuschner and Ellenberg, 2017). Oak forest stands are also promoted in silviculture because they provide high-value timber (Spiecker, 2021). Despite extensive knowledge of the factors that shape forests, our understanding of deciduous forest patches within pine monocultures remains incomplete. The presence of such habitat islands within Scots pine-dominated forest areas may be important for biodiversity, as deciduous forests, in contrast to coniferous forests, provide habitats for the most specialised forest species (Schmidt et al., 2014; Stefańska-Krzaczek et al., 2016). In addition, the rotation period of deciduous stands is longer than that of coniferous stands, giving the forest community more time to develop and allowing older stands to provide more ecosystem services (Jonsson et al., 2020). On the other hand, patch dispersal and fragmentation can have negative effects on species composition (Enoksson et al., 1995; Fletcher et al., 2018). Our study is expected to provide new information on the role of small habitat patches (= habitat islands) for forest biodiversity and to fill this knowledge gap.
The aim of this study was to assess the variability and factors influencing the composition of vascular plant species in old (= mature), managed oak forest stands that are dispersed within Scots pine monocultures. The following research questions were posed: 1) What are the main habitat gradients influencing the vegetation of oak forest patches and which species are indicators of habitat types? 2) Which environmental variables significantly influence species composition and which species traits are associated with these variables?
The study was carried out in a forest complex of the Central European Lowlands located in southwestern Poland, in the Oleśnica Plain and Opole Plain mesoregions (Solon et al., 2018). The study area included forests of four forest districts: Brzeg, Kup, Turawa, and Kluczbork (50.7421°–50.9779° N, 17.4369°–18.2750° E) (Fig. 1). The landscape of the study area was largely shaped by the Oder glaciation. The relatively flat terrain rises from 150 to 200 m above sea level (Kondracki, 2002). The substrate consists mainly of gravel and sand deposits of fluvioglacial and fluvial origin, and clays of glacial origin (Polish Geological Institute, https://geolog.pgi.gov.pl).
The most common soils are podzolic and rusty, while more fertile soils, i.e. brown, black and alluvial, are less common and usually found in deforested areas (Borkowski and Wojniak, 2008). For this reason, compact forest complexes are composed of Scots pine (about 60%), while deciduous forests cover about 30% of the area and are composed mainly of oak, alder, birch, and beech (Zajączkowski et al., 2022).
Hydrological conditions are influenced by the Odra River and its tributaries: Stobrawa, Budkowiczanka, Mała Panew, Nysa Kłodzka and smaller watercourses. The mean annual temperature is 8.3–9.3 ℃, with a gradient from the warmer southwest to the cooler northeast. The mean temperature of the warmest quarter of the year is 18.1–19.0 ℃, and the coldest is −1.2 to −0.4 ℃ (Karger et al., 2017). Annual precipitation ranges from 540 to 696 mm (Karger et al., 2017). The study area is located in the region of Poland with the longest growing season which last more than 220 days (Zielony and Kliczkowska, 2012).
Research plots were selected on the basis of data available in the Forest Data Bank (https://www.bdl.lasy.gov.pl). Using ArcGIS 10.7 software (ESRI, 2022), forest stands located in the districts of Brzeg, Kup, Turawa, and Kluczbork and meeting the following criteria were selected: stand age ≥95 years, proportion of oak (pedunculate and/or sessile) in the stand ≥50%. From the initially pre-selected 477 forest stands (= forest units delimited by the forest management plan), those located in a continuous forest complex under a slight influence of the non-forest surroundings were included in the field study. The field study was conducted in 109 old oak forest stands, in each of which one research plot was established. Data from 92 plots were used for the analysis to avoid excessive plot density (Fig. 1). The uneven distribution of research plots resulted from the actual distribution of stands that met the predefined criteria.
In the selected forest stand, the centre of the plot was located and its geographical coordinates were recorded. A circular plot (r = 8 m, ~200 m2) was then established. In each plot oak trees in the highest layer of the stand were counted and their diameter at breast height was measured. The height of the upper tree layer was measured using an altimeter based on the height of a selected (average) tree. The cover (%) of the following forest layers was estimated for the research plots: T1 – high tree layer, T2 – low tree layer, >8 m and tree crowns below T1 layer, S1 – shrub layer, 3–8 m, S2 – shrub layer, 0.5–3 m, H – herb layer including herb species and tree species <0.5 m in height. For the T-H layers, a floristic survey of all vascular plants was conducted and their cover was estimated using the 7-point Braun-Blanquet scale: r, +, 1, 2, 3, 4, 5 (van der Maarel, 2005). The basic characteristics of the vegetation structure of the research plots are presented in Table S1 (Appendix).
Three datasets were prepared for analysis: 1) plot-species table: presence and cover of species (columns) on the research plots (rows), 2) plot-environment table: environmental variables (columns) describing the research plots (rows), 3) species-traits table: traits (columns) assigned to the recorded species (rows).
As the research plots were selected with a focus on the forest stand composition, the plot-species table included only species in layers S1, S2, and H. Species cover in the dataset was presented in ordinal equivalents 1, 2, 3, 5, 7, 8, 9 for cover degrees r, +, 1, 2, 3, 4, 5 (van der Maarel, 2007). We prepared two variants of the plot-species table: with tree species in separate layers (as recorded in the field) and with tree species merged within the dataset. The merging was done in Juice software (Tichý and Holt, 2006).
In the plot-environment table, each research plot was characterised by: coordinates (latitude, longitude), age of trees (in years), and cover of each tree species in the overstorey (= combined tree layers T1 and T2) for species with a frequency higher than 10%. The number of tree species and overstorey cover were also calculated for each plot. The combined cover was calculated in the Juice software according to a formula proposed by Tichý and Holt (2006). Based on information from the Forest Data Bank, each research plot was also assigned to a Forest Site Type (= Forest Quality Class; a classification unit used in silviculture that mainly describes the soil conditions and potential productivity of forest sites). Based on the Forest Site Types two attributes were assigned to each plot: site productivity and site moisture. Plots were classified as nutrient-poor, intermediate or fertile for site productivity and as fresh or moist for site moisture. Each plot was also assigned attributes describing its position with respect to the complexes of oak forest stands and deciduous stands. All polygons representing old oak forest stands present at a distance of ≤30 m from each other were combined to obtain complexes of old oak forest stands (named: Oak). We calculated the area of these forest complexes in hectares (Oak.area) and their compactness expressed by the circularity ratio (Oak.circularity). The circularity ratio is a measure of the compactness of a shape and is calculated using (4 × π × Area)/(Perimeter2). The resulting dimensionless value ranges from 1 for a circle to 0 for an infinitely long and narrow polygon. All 92 research plots were arranged in such a way that there was only one plot representing productivity and moisture site groups per oak forest complex.
Polygons representing deciduous forest stands (named: Deciduous) were also combined in complexes. Deciduous forest stands were designated on the basis of the percentage of deciduous and coniferous species in a tree layer: forest stands with 0–40% coniferous species were classified as deciduous forest stands. We calculated the area of combined polygons representing deciduous forest stands (Deciduous.area) and their circularity ratio (Deciduous.circularity). Finally, each plot was assigned a specific value of Oak.area, Oak.circularity, Deciduous.area and Deciduous.circularity. The combination of polygons, the calculation of the area and the circularity ratio of the forest complexes were performed using the AcrGiS 10.7 software and the ET GeoWizards toolkit. Each plot was also assigned an altitude above sea level based on the digital terrain model available on the Geoportal website (https://www.geoportal.gov.pl/). All environmental variables assigned to the plots are presented in Table 1.
Variable code | Description |
Stand structure | |
Age.oak | Age of oak trees in forest stands (years) |
T1.2.richness | Species richness (= species number) in overstorey (= combined tree layers T1 and T2) |
T1.2.cover | Cover of overstorey |
Quercus.petraea | Cover of Quercus petraea in overstorey |
Quercus.robur | Cover of Quercus robur in overstorey |
Carpinus.betulus | Cover of Carpinus betulus in overstorey |
Fagus.sylatica | Cover of Fagus sylvatica in overstorey |
Pinus.sylvestris | Cover of Pinus sylvestris in overstorey |
Site description | |
Productivity | Productivity of forest sites based on the unit of the Forest Site Type: 1 – nutrient-poor sites, 2 – intermediate sites, 3 – fertile sites. |
Moisture | Moisture of forest sites based on the unit of the Forest Site Type: 1 – fresh sites, 2 – moist sites. |
Spatial features | |
Oak.area | The area of combined polygons representing old oak forest stands (ha). |
Oak.circularity | Circularity ratio of combined polygons representing old oak forest stands. |
Deciduous.area | The area of combined polygons representing deciduous forest stands. Deciduous forest stands were selected on the basis of the proportion of deciduous and coniferous species. Forest stands with 0–40% coniferous species were assigned as deciduous forest stands. |
Deciduous.circularity | Circularity ratio of deciduous forest stands computed using (4 × π × Area)/(Perimeter2). |
Latitude | Latitude |
Longitude | Longitude |
Altitude | Altitude derived from digital terrain model. |
In the species-traits table the following traits were assigned to the plant species: Category of Forest Species (Heinken et al., 2022), Raunkiaer's life form and Grime's Life Strategy (Pladias https://www.pladias.cz/), and Ecological Indicator Values for moisture, reaction, trophism, light, and temperature (Dengler et al., 2023). In the case of nominal variables, we treated each category as a separate binary variable with 0/1 coding. All species traits assigned to each species are presented in Table 2.
Trait type | Description |
Category of forest species (Heinken et al., 2022) | Forest.species.1.1 – species that can be found mainly in the closed-canopy forest. Forest.species.1.2 – species that occur predominantly along forest edges and in forest openings. Forest.species.2.1 – species that can be equally found in forest and vegetation. Forest.species.2.2 – species that can be found mainly in vegetation, but also in forest. Open.land.species – species of landscape. |
Raunkiaer's life form (Pladias https://www.pladias.cz/) | Phanerophyte – a tree or a shrub, surviving buds at least 30 cm above the ground. Chamaephyte – a herb or a short woody plant with surviving buds above the ground, but not more than 30 cm above it. Hemicryptophyte – a perennial or biennial herb with surviving buds on aboveground shoots at the level of the ground. Geophyte – a perennial plant with surviving buds below ground, usually with bulbs, tubers or rhizomes. Therophyte – an annual herb that survives the unfavourable season only as seeds. |
Grime's life strategy (Pladias https://www.pladias.cz/) | Strategy.C – competitor Strategy.CR – competitor/ruderal Strategy.CS – competitor/stress-tolerator Strategy.CSR – competitor/stress-tolerator/ruderal Strategy.R – ruderal Strategy.S – stress-tolerator Strategy.SR – stress-tolerator/ruderal |
Ecological Indicator Values (Dengler et al., 2023) | Numerical indicator values for niche position for moisture, reaction, nitrogen/trophism, light, and temperature. |
The data were analysed in the R software environment (R Core Team, 2022). We analysed the general variability of species composition for the research plots based on the plot-species table with tree species in separate layers using DCA ordination (axis 1 length = 5.2, see Table S2 in the Appendix). Ordination was performed using the “vegan” package and the decorana() function. Productivity and moisture from the plot-environment table were used as grouping variables in the ordination plots.
For forest stands on sites with different productivity (nutrient-poor sites, intermediate sites, fertile sites) and moisture (fresh sites, moist sites), we calculated a species indicator value and p-value using the indval() function of the “labdsv” package. This analysis showed whether the studied flora included species associated with forest stand groups on the sites characterised by different productivity and moisture.
The variability of species composition in the studied patches of old oak forests was also assessed using Whittaker's index of beta diversity (= species turnover, βw) (Koleff et al., 2003). This index is based on the presence/absence of species, so we used the plot-species table with tree species merged within the dataset. The analysis was performed in the “vegan” package using the betadiver() function. First, the βw index was calculated for all plots, as well as the indices for productivity and moisture groups, i.e. nutrient-poor sites, intermediate sites, fertile sites, fresh sites, and moist sites. In these groups, the index for pairs of plots and then the minimum, maximum and mean βw values for the group were calculated. Differences between means were tested using the Kruskal-Wallis and Dunn tests for productivity groups, and the Mann-Whitney test for moisture groups. Next, the βw index was calculated between the productivity groups and between the moisture groups, and between these groups and all plots, i.e. the total flora.
RLQ analysis was used to show the relationships between species composition and environmental variables. This is a three-table ordination method that allows analysis of the plot-environment table (R), plot-species table (L), and species-traits table (Q) to show the main patterns of co-variation between environmental variables and trait data (Dolédec et al., 1996). For this analysis we used the plot-species table with tree species merged within the dataset. The analysis was performed using the “ade4” package following the procedure proposed by other authors (Dolédec et al., 1996; Kleyer et al., 2012). First, we performed a correspondence analysis (CA) for the plot-species table using the dudi.coa() function and a principal component analysis (PCA) for the plot-environment and species-traits tables using the dudi.pca() function, followed by an RLQ analysis using the rlq() function. The significance of the model was tested using the randtest() function. Relationships between environmental variables and species traits were analysed using the fourthcorner() and p.adjust.4thcorner() functions, and relationships with the RLQ axes were examined using the fourthcorner.rlq() function.
Based on the species scores on the first two axes of the RLQ analysis, we performed classification to obtain functional species groups based on traits. We used the hclust() function with Ward's hierarchical clustering for classification. Next, we used the Calinski-Harabasz criteria to find the best partition (between 2 and 6 groups) and found the interpretation of the groups in terms of species traits (Kleyer et al., 2012).
A total of 194 vascular plant species (from 5 to 53 species per plot, mean 22) were found in the plant communities of the studied oak forests. Differences in species composition were found between nutrient-poor and fertile sites, and between fresh and moist sites (Fig. 2). A clear transition from forests growing on nutrient-poor and fresh sites to forests growing on fertile and moist sites was identified along the first DCA axis. However, plots representing specific productivity and moisture groups did not form separate groups on the ordination plot.
Fertile sites had 27 indicator species in the herb and shrub layers (Table S3 in the Appendix), 12 more than on nutrient-poor sites (15 species). Among the productivity groups, intermediate sites had the lowest number of indicator species (2). Species with the highest indicator value for fertile sites (>0.20, listed in descending order) were: Carpinus betulus (S2 and H layer), Carex remota, Maianthemum bifolium, Fraxinus excelsior (H), Quercus robur, Lamium galeobdolon, Fraxinus excelsior (S2), Anthyrium filix-femina, Cicerbita muralis, Polygonatum multiflorum, and Carex sylvatica. Species with the highest indicator value for nutrient-poor sites were Vaccinium myrtillus, Pinus sylvestris (H), Avenella flexuosa, Quercus petraea (H, S1, and S2), and Calamagrostis arundinacea.
Moist sites had 27 indicator species, 17 more than on fresh sites (10 species). Species with the highest indicator value for moist sites (>0.20, listed in descending order) were Carpinus betulus (S2), Quercus robur (H), Carpinus betulus (S1), Lysimachia vulgaris, Dryopteris carthusiana, Deschampsia cespitosa, Maianthemum bifolium, Frangula alnus (H), Carex remota, Oxalis acetosella, Polygonatum multiflorum, Athyrium filix-femina, Juncus effusus, and Carex sylvatica. Considering fresh sites, highest indicator values were found for Quercus petraea (H), Vaccinium myrtillus, Carex pilulifera, Pinus sylvestris (H), Calamagrostis arundinacea, Fagus sylvatica (S2), Avenella flexuosa, and Pteridium aquilinum. Fertile and moist sites shared 15 indicator plant species, while nutrient-poor and fresh sites shared 7 species.
The differences between the mean Whittaker's indices of beta diversity (βw) were not large, but statistically significant (Table 3). Among the groups representing productivity, the βw index was lowest for nutrient-poor sites and highest for fertile sites. Between groups representing moisture, moist sites were more diverse than fresh sites. The greatest floristic differences were found between nutrient-poor and fertile sites. Moreover, nutrient-poor sites differed most strongly from the total flora (flora of all plots) and moist sites differed least from the total flora.
In the RLQ model, the first axis explained 58.03% of the variance, and the second axis explained 37.14% of the variance (95.17% in total) (Table 4). The results of the RLQ models were significant (p = 0.01) (Table S4 in the Appendix). In case of environmental variables, the first RLQ axis represented a transition from forests with older trees, with a more abundant and richer overstorey layer and with a significant proportion of Quercus robur and Carpinus betulus, to forests with a proportion of Quercus petraea in deciduous forest complexes with greater circularity (Fig. 3 and Tables S5–S7, Fig. S1 in the Appendix). The second axis additionally indicated geographical (longitudinal) and altitudinal gradients. In case of species traits, preferences for high soil reaction and trophism were negatively correlated with the first axis, and a preference for light availability was positively correlated with the first axis. The second axis separated species of closed (1.1) and open forests (2.1, 2.2) and species with different preferences for soil moisture.
Total inertia = 1.273 | |||
Eigenvalues | Axis 1 | Axis 2 | |
Eigenvalue | 0.73887 | 0.47283 | |
Projected inertia | 58.0299% | 37.1353% | |
Cumulative projected inertia | 58.03% | 95.17% | |
Covariance | 0.8595731 | 0.6876233 | |
Correlation | 0.3527418 | 0.2553948 | |
Inertia and coinertia | Inertia | Max | Ratio |
R Axis 1 | 2.516267 | 3.636706 | 0.6919081 |
R Axis 1 & Axis 2 | 5.868958 | 6.207810 | 0.9454151 |
Q Axis 1 | 2.359908 | 3.527430 | 0.6690163 |
Q Axis 1 & Axis 2 | 4.522046 | 6.520042 | 0.6935609 |
Correlation L | Corr. | Max | Ratio |
Axis 1 | 0.3527418 | 0.7051465 | 0.5002390 |
Axis 2 | 0.2553948 | 0.5805991 | 0.4398816 |
Explanations: In the eigenvalues section, there is the decomposition of total inertia by axes 1 and 2. In the inertia and co-inertia section we present the inertia found in each RLQ axis, the maximum inertia found in the original ordination of each table, and the ratio which returns the variance recovered of the R (environment) and Q (traits) table by the RLQ axis. The correlation L showed the comparison between trait-based species scores and the environmental site scores found by the RLQ analysis, to the maximum possible value (max) resulting from the correspondence analysis of the L (species) table. |
We identified 48 (23 positive and 25 negative) significant associations between environmental variables and species traits for single comparisons (Fig. 4a, Table S8 in the Appendix). Environmental variables were mainly related to forest species categories and species ecological requirements. After taking into account interactions between variables, we identified 18 (11 positive and 7 negative) significant associations (Fig. 4b and Table S8 in the Appendix).
There was a positive relationship between species of the closed-canopy forest (1.1) and overstorey richness and cover, Carpinus betulus cover, and deciduous forest area. Species with higher requirements for moisture, nitrogen and high reaction were positively related to stand age. Species with higher moisture requirements were also positively related to Quercus robur cover and longitude. Species preferring high soil reaction were positively correlated with Carpinus betulus cover. Species with high light requirements were positively related to the circularity of deciduous forest complexes.
Negative relationships were found between species of moist, fertile and high reaction sites and Quercus petraea cover, and between light-demanding species and overstorey richness and cover, Carpinus betulus cover, and area of deciduous forest complexes.
We identified three functional groups of species (Fig. 5 and Fig. S2, Fig. S3 in the Appendix). Group A was distinguished by species of closed-canopy forests (F.1.1), and groups B and C by species of open forests (2.1 and/or 2.2) (Figs. S4–S7 in the Appendix). Additionally, group C was distinguished by the presence of open landscape species (O). Group A was distinguished by phanerophytes and geophytes, while group B was distinguished by hemicryptophytes. Groups A and B were distinguished by the presence of species preferring fertile habitats with high soil reaction, while groups B and C included species with high light requirements. Species with high moisture requirements were also found in group B. Life strategies did not significantly define the groups.
We found that the species composition of the studied old managed oak stands was diverse, corresponding with the environmental gradient, from nutrient-poor to fertile sites and from fresh to moist sites. This indicates that, despite the pressure caused by forest management and the resulting structural distortions (Burrascano et al., 2018), site conditions remain the key determinant of species composition (Wei et al., 2020). Variability in species composition associated with habitat variability has also been observed in Scots pine monocultures surrounding patches of deciduous stands (Stefańska-Krzaczek et al., 2019; Stefańska-Krzaczek and Pech, 2014). However, Scots pine monocultures on fertile and intermediate sites represent disturbed plant communities and are typically secondary vegetation for deciduous forests (Stefańska-Krzaczek and Pech, 2014). Oak stands can form natural communities in oligotrophic, mesotrophic, and eutrophic habitats, as well as in habitats with different moisture conditions (Kącki et al., 2013, 2016; Matuszkiewicz, 2001). Differences in the species composition of the oak forest stands studied show that plant communities associated with oak islands can be classified into acidophilous oak forests, oak-hornbeam forests, and riparian forests (Kącki et al., 2013). Therefore, we found that the variability observed in the species composition of the oak forest stands studied is consistent with the natural variability of oak forests. This is in line with the results of previous studies which showed that the oldest managed stands are usually characterised by the species composition of specific forest types (Krzaczek and Stefańska-Krzaczek, 2022). The species pool and species richness of the analysed oak stands are not high (194 species, mean 22) compared to well-preserved patches of different forest types formed by oaks (Kącki et al., 2016; Matuszkiewicz, 2001). However, we conclude that the most important role of the studied oak stands is to increase the diversity of forest mosaic, not only because of the distinctive species composition of the overstorey, but also because of the structure of the entire plant community. We also found that indicator species are not only local indicators of the analysed habitats, but their indicator role is known on a broader scale (Kącki et al., 2013, 2016).
Vascular plants found in the studied forests are species common to different types of forest vegetation (Kącki et al., 2013), and some of them also colonise Scots pine monocultures (Stefańska-Krzaczek et al., 2019; Stefańska-Krzaczek and Pech, 2014). This means that the habitat islands studied are not isolated from the surrounding area. This supports previous conclusions from studies of habitat islands, indicating that some species frequently colonise the area around such islands, even if it is formed by alien plant species (Horák et al., 2019a). The species turnover between habitat islands and their surroundings also shows a good vegetation status of Scots pine forests, as no similarity was found between typical coniferous plantations and semi-natural deciduous forests (Coote et al., 2012).
Our research confirms previous reports that stand structure has a significant influence on the lower forest layers (Augusto et al., 2003; Barbier et al., 2008). For the oak stands studied, we found that the richness and cover of the overstorey determined the presence of closed forest species. This confirms previous observations that forests with a richer overstorey, such as oak-hornbeam forests, favour specialised forest species (Stefańska-Krzaczek et al., 2016). Furthermore, tree cover reduces light intensity and shapes the microclimate (Kovács et al., 2017), which is beneficial for the occurrence of forest specialists (Härdtle et al., 2003; Tinya et al., 2009).
We found that the species composition of the understorey in the studied forests depended primarily on the proportion of pedunculate or sessile oak in the stand. Pedunculate oak has slightly higher requirements for soil fertility and moisture (Eaton et al., 2016) and in managed forests is introduced on soils that are heavier, richer in nutrients and better hydrated than sessile oak habitats. For this reason, the proportion of Quercus robur in forest stands is associated with understorey species that require higher soil moisture and, at the same time, with species of more fertile and alkaline habitats. Moreover, the rotation period is longer on fertile sites, as this allows for maximum profitability (Nakajima et al., 2017). Therefore, pedunculate oak stands are different in terms of species composition because they are characterised by specific soil conditions and they are also older, which explains the dependence of species composition on stand age found in our study. Considering the specificity of the analysed forest patches, it should be emphasised that the relationship between species composition and stand age mainly reflects stand composition (the proportion of Q. robur) which is selected according to site characteristics. Although the composition of the understorey should change with stand age, this trend is mainly observed in a long-term perspective, e.g. during the whole rotation period (Moola and Vasseur, 2004; Stefańska-Krzaczek et al., 2019). Most of the analysed stands are between 100 and 150 years old, and a difference of 50 years may not be sufficient to detect differences in species composition (Duguid and Ashton, 2013).
Sessile oak is more tolerant of drought (Eaton et al., 2016), so it usually grows well in managed forests established on poorer and drier sites. For this reason we found a relationship between the presence of Q. petraea and species with lower soil moisture, fertility and reaction requirements. These forest stands are also younger, as increasing the harvest age on poorer sites does not increase forest profitability (Nakajima et al., 2017).
Among the stand characteristics, we also found that the proportion of hornbeam was important for the species composition of the understorey. Carpinus betulus is an indicator species for fertile but not moist habitats (Kącki et al., 2013; Matuszkiewicz, 2001) and is therefore associated with species that prefer high soil reaction and nutrient-rich habitats. Hornbeam in a tree stand also reduces light penetration and limits the growth of light-demanding species (Kwiatkowska et al., 1997), which is reflected in the identified negative correlation between the presence of this species in a tree stand and plants with high light requirements.
Other variables with a significant effect on species composition were longitude and altitude. The location of vegetation patches and topography are important for species composition, especially in areas with a clearly diversified landscape (Bátori et al., 2023; Sewerniak and Puchałka, 2020). Our study shows that the location of vegetation patches is also important in areas with low diversity of geomorphological features. Patches in different locations may differ in terms of environmental conditions, which expands the total pool of forest species, e.g. in the study area, the proportion of species with higher moisture requirements increased with longitude. Our study also revealed the significant effect of the area of deciduous forest complexes, i.e. positive for close-forest species and negative for species with high light requirements. In general, light availability is a very important factor shaping the structure and diversity of the understorey (Dormann et al., 2020; Plue et al., 2013; Tinya et al., 2009). Mixed deciduous stands are more shaded than pine forests (Lieffers et al., 1999; Sercu et al., 2017), and insufficient light condition initially limits light-demanding species (Plue et al., 2013). In small areas, light availability is better at the edge of the patch, while in larger areas shading is more uniform, hence the negative correlation between light-demanding species and the area of deciduous stands. However, the presence of species with high light requirements was correlated with the circularity of deciduous forest stands. This can be explained by the fact that smaller and more sunny patches of deciduous forests tend to have a more regular shape than complexes occupying larger areas. Heterogeneous light conditions in the analysed forest stands have a positive effect by promoting the survival of closed forest species, but also by favouring light-demanding species which are also an important component of forest biodiversity (Kozel et al., 2021).
Managed forests cover a significant area, and for this reason they should be recognised as the most important habitats for forest species, especially because sustainable forest management promotes rather than reduces biodiversity (Schulze, 2018). Deciduous forests are important ecosystems for the conservation of forest flora diversity (Hermy et al., 1999; Schmidt et al., 2014; Stefańska-Krzaczek et al., 2016), and the presence of oaks in the stand is positively correlated with species richness (Zeller et al., 2023). Differences in the species composition of the vegetation resulting from the diversity of habitats indicate that the studied oak stands are not just patches of trees different from those found in the monocultures dominating the surrounding area, but above all they are distinct plant communities adapted to the habitat conditions. Since the fact that deciduous stands provide more favourable conditions for species of closed-canopy forests or ancient forests than coniferous ones (Schmidt et al., 2014; Stefańska-Krzaczek et al., 2016, 2022), the forest stands analysed in our study add new value to forest complexes dominated by Scots pine monocultures. Our results are in line with observations of other authors which indicate that habitat islands of deciduous forests have a positive effect on the biodiversity of forest complexes (Horák et al., 2019a, 2019b; Kennedy and Spies, 2005). The preservation of such patches is important for various groups of organisms that prefer the oldest stands (Hofmeister et al., 2015; Vandekerkhove et al., 2013). This is due to the fact that old tree stands create a specific microclimate, but their sensitivity to management should also be taken into account (Kovács et al., 2017, 2020; Máliš et al., 2023). It is then important to preserve as many patches of old oak forests as possible, as each of them, even small ones, can make a significant contribution to the biodiversity of the forest complex (Fahrig, 2017; Fahrig et al., 2019; Honnay et al., 1999; Lindenmayer et al., 2006).
Patches of deciduous stands are important for increasing diversity and should be preserved in forest complexes as they expand ecosystem services similar to mixed-species stands (Felton et al., 2010; Huuskonen et al., 2021; Juchheim et al., 2020).
Ewa Stefańska-Krzaczek: Writing – review & editing, Writing – original draft, Visualization, Supervision, Methodology, Investigation, Funding acquisition, Formal analysis, Data curation, Conceptualization. Rafał Krzaczek: Writing – review & editing, Methodology, Formal analysis, Data curation. Natalia Mazurek: Writing – review & editing, Writing – original draft, Visualization, Investigation. Damian Chmura: Writing – review & editing, Methodology, Formal analysis.
The authors declare the following financial interests/personal relationships which may be considered as potential competing interests:
Ewa Stefanska-Krzaczek reports financial support was provided by National Science Centre Poland (Narodowe Centrum Nauki). If there are other authors, they declare that they have no known competing financial interests or personal relationships that could have appeared to influence the work reported in this paper.
The authors would like to thank the Regional Directorate of State Forests in Katowice and the managers and workers of Brzeg, Kup, Turawa and Kluczbork forest districts for their acceptance of field research.
Supplementary data to this article can be found online at https://doi.org/10.1016/j.fecs.2024.100235.
1. | Yongkang Lai, Xihan Mu, Dasheng Fan, et al. Methodology comparison for correcting woody component effects in leaf area index calculations from digital cover images in broadleaf forests. Remote Sensing of Environment, 2025, 321: 114659. DOI:10.1016/j.rse.2025.114659 |
2. | Sima Lotfi Asl, Iraj Hassanzad Navroodi, Aman Mohammad Kalteh. Sensitivity analysis and performance evaluation of neural networks for predicting forest stand volume - A case study: District 2, Kacha, Guilan province, Iran. Journal of Forest Science, 2024, 70(5): 209. DOI:10.17221/111/2023-JFS |
3. | Tereza Pohanková, Vilém Pechanec. Assessing the Cooling Potential of Vegetation in a Central European Rural Landscape: A Local Study. Land, 2024, 13(10): 1685. DOI:10.3390/land13101685 |
4. | Meiling Liu, Ge Chen, Yanan Wen, et al. Combining radiative transfer model with statistical model for distinguishing heavy metal stress level in rice from Sentinel-2 satellite images. Journal of Applied Remote Sensing, 2024, 18(04) DOI:10.1117/1.JRS.18.044516 |
5. | Edney L. da Vitória, André O. Nardotto Júnior, Luis F. O. Ribeiro, et al. Leaf area estimation in Coffea canephora genotypes by neural networks and multiple regression. Revista Brasileira de Engenharia Agrícola e Ambiental, 2024, 28(9) DOI:10.1590/1807-1929/agriambi.v28n9e279246 |
6. | Dilek Soysal. A Non-destructive Leaf Area Prediction Model and Some Physical Leaf Properties in Apples. Applied Fruit Science, 2024, 66(4): 1181. DOI:10.1007/s10341-024-01103-x |
7. | John W. Smith, R. Quinn Thomas, Leah R. Johnson. Parameterizing Lognormal state space models using moment matching. Environmental and Ecological Statistics, 2023, 30(3): 385. DOI:10.1007/s10651-023-00570-x |
8. | Sinan Bulut, İbrahim Aytaş. Modeling potential distribution and above-ground biomass of Scots pine (Pinus sylvestris L.) forests in the Inner Anatolian Region, Türkiye. Environmental Monitoring and Assessment, 2023, 195(12) DOI:10.1007/s10661-023-12101-z |
9. | Md Nasim Reza, Milon Chowdhury, Sumaiya Islam, et al. Leaf Area Prediction of Pennywort Plants Grown in a Plant Factory Using Image Processing and an Artificial Neural Network. Horticulturae, 2023, 9(12): 1346. DOI:10.3390/horticulturae9121346 |
10. | Tomasz Trzepieciński, Krzysztof Szwajka, Marek Szewczyk. An Investigation into the Friction of Cold-Rolled Low-Carbon DC06 Steel Sheets in Sheet Metal Forming Using Radial Basis Function Neural Networks. Applied Sciences, 2023, 13(17): 9572. DOI:10.3390/app13179572 |
11. | Sinan BULUT, Alkan GÜNLÜ, Sedat KELES. Assessment of the interactions among net primary productivity, leaf area index and stand parameters in pure Anatolian black pine stands: A case study from Türkiye. Forest Systems, 2023, 32(1): e003. DOI:10.5424/fs/2023321-19615 |
12. | Gabriela Cristina Costa Silva, Júlio César Lima Neves, Gustavo Eduardo Marcatti, et al. Improving 3-PG calibration and parameterization using artificial neural networks. Ecological Modelling, 2023, 479: 110301. DOI:10.1016/j.ecolmodel.2023.110301 |
13. | Mehmet Seki. Predicting stem taper using artificial neural network and regression models for Scots pine (Pinus sylvestrisL.) in northwestern Türkiye. Scandinavian Journal of Forest Research, 2023, 38(1-2): 97. DOI:10.1080/02827581.2023.2189297 |
14. | Juntao Gu, Zhongbin Su, Rui Gao, et al. Improving QGA-ELM Inversion Model of Rice Leaf Area Index Based on UAV Remote Sensing Image. Mobile Information Systems, 2022, 2022: 1. DOI:10.1155/2022/9658966 |
15. | Y Karatepe, MJ Diamantopoulou, R Özçelik, et al. Total tree height predictions via parametric and artificial neural network modeling approaches. iForest - Biogeosciences and Forestry, 2022, 15(2): 95. DOI:10.3832/ifor3990-015 |
16. | Jehad Mahmoud Hussein Ighbareyeh, Ana Cano-Ortiz, Eusebio Cano. Phytosociology and Vegetation of Plants of Beit Jibrin in Palestine. Land, 2022, 11(2): 264. DOI:10.3390/land11020264 |
17. | Alkan Günlü, İlker Ercanlı, Muammer Şenyurt, et al. Estimation of some stand parameters from textural features from WorldView-2 satellite image using the artificial neural network and multiple regression methods: a case study from Turkey. Geocarto International, 2021, 36(8): 918. DOI:10.1080/10106049.2019.1629644 |
18. | Pábulo Diogo de Souza, Carlos Alberto Araújo Júnior, Christian Dias Cabacinha, et al. PERFORMANCE DA MODELAGEM PARA CLASSIFICAÇÃO DE SÍTIOS FLORESTAIS EM BASES DE DADOS COM OUTLIERS. Nativa, 2021, 9(1): 54. DOI:10.31413/nativa.v9i1.11202 |
19. | Sinan BULUT, Alkan GÜNLÜ, Mucahit Yılmaz SÖNMEZ. Saf Karaçam Meşcerelerinde Yaprak Alan İndeksi ile Meşcere Parametreleri Arasındaki İlişkilerin Modellenmesi. Bartın Orman Fakültesi Dergisi, 2021, 23(3): 980. DOI:10.24011/barofd.1002569 |
20. | E Asriani, Robika. Application of artificial neural network with backpropagation algorithm for estimating leaf area. IOP Conference Series: Earth and Environmental Science, 2020, 599(1): 012046. DOI:10.1088/1755-1315/599/1/012046 |
21. | Jie Zou, Wei Hou, Ling Chen, et al. Evaluating the impact of sampling schemes on leaf area index measurements from digital hemispherical photography in Larix principis-rupprechtii forest plots. Forest Ecosystems, 2020, 7(1) DOI:10.1186/s40663-020-00262-z |
22. | Ahmet Öztürk, Bilal Cemek, Hüsnü Demirsoy, et al. Modelling of the leaf area for various pear cultivars using neuro computing approaches. Spanish Journal of Agricultural Research, 2020, 17(4): e0206. DOI:10.5424/sjar/2019174-14675 |
23. | Ramazan Ozçelik, Onur ALKAN, HASAN ALKAN. Development of regional stem taper models for some important tree species of Turkey: Case study of Bucak. Turkish Journal of Forestry | Türkiye Ormancılık Dergisi, 2019. DOI:10.18182/tjf.656457 |
24. | Alkan Günlü, Sedat Keleş, İlker Ercanli, et al. Spatial Modeling in Forest Resources Management. Environmental Science and Engineering, DOI:10.1007/978-3-030-56542-8_16 |
Variable code | Description |
Stand structure | |
Age.oak | Age of oak trees in forest stands (years) |
T1.2.richness | Species richness (= species number) in overstorey (= combined tree layers T1 and T2) |
T1.2.cover | Cover of overstorey |
Quercus.petraea | Cover of Quercus petraea in overstorey |
Quercus.robur | Cover of Quercus robur in overstorey |
Carpinus.betulus | Cover of Carpinus betulus in overstorey |
Fagus.sylatica | Cover of Fagus sylvatica in overstorey |
Pinus.sylvestris | Cover of Pinus sylvestris in overstorey |
Site description | |
Productivity | Productivity of forest sites based on the unit of the Forest Site Type: 1 – nutrient-poor sites, 2 – intermediate sites, 3 – fertile sites. |
Moisture | Moisture of forest sites based on the unit of the Forest Site Type: 1 – fresh sites, 2 – moist sites. |
Spatial features | |
Oak.area | The area of combined polygons representing old oak forest stands (ha). |
Oak.circularity | Circularity ratio of combined polygons representing old oak forest stands. |
Deciduous.area | The area of combined polygons representing deciduous forest stands. Deciduous forest stands were selected on the basis of the proportion of deciduous and coniferous species. Forest stands with 0–40% coniferous species were assigned as deciduous forest stands. |
Deciduous.circularity | Circularity ratio of deciduous forest stands computed using (4 × π × Area)/(Perimeter2). |
Latitude | Latitude |
Longitude | Longitude |
Altitude | Altitude derived from digital terrain model. |
Trait type | Description |
Category of forest species (Heinken et al., 2022) | Forest.species.1.1 – species that can be found mainly in the closed-canopy forest. Forest.species.1.2 – species that occur predominantly along forest edges and in forest openings. Forest.species.2.1 – species that can be equally found in forest and vegetation. Forest.species.2.2 – species that can be found mainly in vegetation, but also in forest. Open.land.species – species of landscape. |
Raunkiaer's life form (Pladias https://www.pladias.cz/) | Phanerophyte – a tree or a shrub, surviving buds at least 30 cm above the ground. Chamaephyte – a herb or a short woody plant with surviving buds above the ground, but not more than 30 cm above it. Hemicryptophyte – a perennial or biennial herb with surviving buds on aboveground shoots at the level of the ground. Geophyte – a perennial plant with surviving buds below ground, usually with bulbs, tubers or rhizomes. Therophyte – an annual herb that survives the unfavourable season only as seeds. |
Grime's life strategy (Pladias https://www.pladias.cz/) | Strategy.C – competitor Strategy.CR – competitor/ruderal Strategy.CS – competitor/stress-tolerator Strategy.CSR – competitor/stress-tolerator/ruderal Strategy.R – ruderal Strategy.S – stress-tolerator Strategy.SR – stress-tolerator/ruderal |
Ecological Indicator Values (Dengler et al., 2023) | Numerical indicator values for niche position for moisture, reaction, nitrogen/trophism, light, and temperature. |
Total inertia = 1.273 | |||
Eigenvalues | Axis 1 | Axis 2 | |
Eigenvalue | 0.73887 | 0.47283 | |
Projected inertia | 58.0299% | 37.1353% | |
Cumulative projected inertia | 58.03% | 95.17% | |
Covariance | 0.8595731 | 0.6876233 | |
Correlation | 0.3527418 | 0.2553948 | |
Inertia and coinertia | Inertia | Max | Ratio |
R Axis 1 | 2.516267 | 3.636706 | 0.6919081 |
R Axis 1 & Axis 2 | 5.868958 | 6.207810 | 0.9454151 |
Q Axis 1 | 2.359908 | 3.527430 | 0.6690163 |
Q Axis 1 & Axis 2 | 4.522046 | 6.520042 | 0.6935609 |
Correlation L | Corr. | Max | Ratio |
Axis 1 | 0.3527418 | 0.7051465 | 0.5002390 |
Axis 2 | 0.2553948 | 0.5805991 | 0.4398816 |
Explanations: In the eigenvalues section, there is the decomposition of total inertia by axes 1 and 2. In the inertia and co-inertia section we present the inertia found in each RLQ axis, the maximum inertia found in the original ordination of each table, and the ratio which returns the variance recovered of the R (environment) and Q (traits) table by the RLQ axis. The correlation L showed the comparison between trait-based species scores and the environmental site scores found by the RLQ analysis, to the maximum possible value (max) resulting from the correspondence analysis of the L (species) table. |